Geothermal energy looks to be a global energy game-changer — and Cockrell School researchers are major players in advancing the technology.
by Stacia Miller
Nearly 3,000 kilometers below Earth’s surface, unstable isotopes fling stray neutrons into a primordial soup of molten iron and nickel. As they transform into more stable versions of themselves, the isotopes emit tremendous amounts of heat, helping to keep the Earth’s core at a toasty 5,000 or so degrees Celsius. That heat continuously radiates outward, melting igneous and metamorphic rocks in the mantle that in turn boil underground aquifers and char rock formations in the crust.
No, this isn’t the trailer for Hollywood’s new sci-fi thriller — it’s the reason geothermal could be a global energy game-changer.
Heat from the Earth’s core is ubiquitous and constant. Energy produced from that heat isn’t dependent on whether it’s sunny or windy outside. It’s significantly cleaner than coal and other fossil fuels because it releases primarily water vapor into the air. And geothermal power plants typically have a smaller footprint than their solar, wind and coal counterparts.
By 2050, geothermal energy could provide electricity for more than 65 million U.S. homes, as well as heat and cool another 28 million, according to the Department of Energy’s (DOE) Geothermal Technologies Office. If the technology grows as quickly as some of DOE’s estimates, the resulting emissions reduction would be equivalent to 26 million fewer cars on U.S. roads each year. If geothermal power constitutes just 3% of the global energy mix in 2050, as much as 9 gigatons of greenhouse gas emissions could be eliminated.
There’s one big challenge, though — safely and efficiently harnessing the heat. The deepest humans have ever drilled into the Earth is a mere 12 kilometers. At that depth, equipment can melt, corrode or get stuck. Concrete can soften, causing the pipes inside to shift and sway. There’s the risk of induced seismicity. And it’s all incredibly expensive.
“Geothermal energy has tremendous potential, but it comes with tremendous challenges. We have to prove that it can be economically viable and can generate power at levels comparable to other energy sources — and those are not trivial problems.”
— Mukul Sharma, professor, Hildebrand Department of Petroleum and Geosystems Engineering (UT PGE)
“Geothermal is not yet competitive with other kinds of energy, and that’s holding it back,” agrees UT PGE professor Eric van Oort, “To put it simply, we need to learn to drill a lot deeper and faster in hotter, harder rock.”
Enter Sharma, van Oort and fellow Cockrell School of Engineering researchers. With more than 30 centers, institutes, affiliate programs and research groups working in energy, the Cockrell School is at the forefront of solving complex energy challenges like those that geothermal presents.
Say Yes to EGS
Sharma and his team are working to identify best practices in what is known as Enhanced Geothermal Systems (EGS). For geothermal energy to be harnessed successfully, three things must be present: heat, fluid and space for that fluid to circulate through hot rocks in the subsurface. These three criteria are easily met in places like Iceland, Yellowstone National Park and the Pacific Ring of Fire, where steam rises to the surface on its own and is easily captured from hot springs, geysers and fumaroles. The rest of the world? Not so much.
In an EGS, scientists add whatever elements are missing from the equation. For Sharma’s team, that means modeling the most effective way to introduce fractures into a geothermal system so that engineers can put fluid into an injection well, track the fluid as it travels through and is heated by hot rock via those manmade fractures and maximize the amount that reaches a nearby production well. Once the hot fluid is captured in the production well, it can be pumped to the surface to power a turbine and generate electricity.
Sharma’s work is funded by a $4 million DOE grant, part of more than $46 million awarded for research at its Frontier Observatory for Research in Geothermal Energy (FORGE) site in Utah. To date, Sharma and graduate assistants Meng Cao and Qianjun Liu have designed and refined eight potential fracture models for transferring fluid between two specific wells, dubbed 16A and 16B, at the FORGE site. Sharma oversaw fractures based on the first two models over the summer. Fractures based on the remaining six models will take place early next year.
An example of Enhanced Geothermal Systems fracture models.
Engineers at the site can monitor post-fracture activity — how fast and far the fluid is moving and how much of what is injected via 16A makes its way to 16B — thanks to graduate research assistant Yuhao Ou. With Sharma’s guidance, Ou created a model outlining the best way to install three strings of fiber-optic cable in 16B to track temperature, rock strain and deformation and seismic vibrations.
“It’s 220 degrees Celsius in these wells. Oil and gas wells have run fiber in lower temps, but doing this in a geothermal well requires a much more robust fiber, careful planning and great attention to detail,” says Sharma, who is also co-founder of EGS company Geothermix. “Our FORGE installation is one of the first to be successful, and whatever we learn from the data relayed by these cables over the course of the FORGE project can be applied across the industry.”
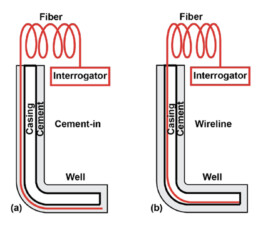
A model for installing fiber-optic cables in wells.
Another application coming out of Sharma’s labs may skip over all the geothermal challenges entirely. Sharma and graduate research assistant Ziming Jing have developed a portable generator that uses hot water and steam generated at existing oil and gas wells to produce energy. Jing’s prototype transfers hot and cold fluid through a series of alternating plates using thermoelectric chips. The chips generate a voltage that transfers the heat to one end of the device, which is then used to produce energy on site.
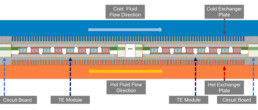
A model of a portable generator that could solve many geothermal energy challenges.
The biggest benefit, says Sharma, is that the hot water used to generate power comes from oil and gas assets that already exist.
“You don’t need to drill geothermal wells at all — you can go to an existing facility that already has hot water and use that to power the generator,” he says. “It saves a lot of money. There are no moving parts. It’s highly mobile.”
And it’s sitting in the Chemical and Petroleum Engineering building (CPE) basement, all ready to go. Sharma plans to test the prototype early next year at an East Texas oilfield. If it performs as simulations indicate, it could eventually be scaled up for industrial use at refineries or consumer use in homes.
No Stress, AGS/CLGS
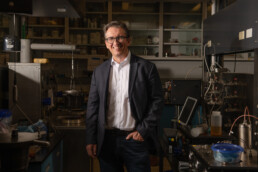
Petroleum and geosystems engineering professor Eric van Oort.
Another promising method for capturing geothermal energy where there’s a dearth of fluid and permeability is to drill and install a u-shaped closed-loop well system. Fluid travels through a radiator-like network of horizontal pipes deep underground so that it can absorb as much heat as possible from the surrounding hot rock.
When the hot fluid travels back to the surface it can be used as an energy source for direct heating or generating electricity. The fluid can be pumped, but may also circulate on its own in a self-sustained way using what is known as the “thermosiphon” effect, when temperature impacts fluid density. These close-looped designs are called Advanced Geothermal Systems (AGS) or Closed-Loop Geothermal Systems (CLGS), and are the focus of van Oort’s geothermal research efforts.
The most obvious benefit of AGS is that nothing is actually injected into the subsurface. All fluid circulates through closed wells, so no natural or manmade fractures are necessary. Not only does this eliminate the risks of induced seismicity, downhole fluid losses and greenhouse gas emissions, it also makes AGS a viable option in states and countries (read: most of Europe) where fracking is not allowed.
Even still, AGS faces many of the same engineering problems as EGS. Van Oort’s Rig Automation Performance Improvement in Drilling group (RAPID) is working on nearly all of them.
“We have the most all-encompassing geothermal drilling research program, and we are systematically tackling pain points, relevant technological gaps and needs” says van Oort.
Those pain points include low hole-making efficiency in hard rocks such as granites and basalts, high temperature, lost circulation, drilling failures such as stuck pipe, and ineffective casing and cementing. These problems also exist in the oil and gas industry, but have been (and continue to be) mitigated by more than a century of technological advancements. While many of those techniques can be applied to geothermal wells, what works in oil and gas doesn’t always neatly translate to higher temperature, increased depth and harder rock. But it’s a start.
Case in point: PGE research scientist Ningyu Wang and Bureau of Economic Geology research associate Mohamed Shafik Khaled have modified existing petroleum industry temperature-calculation models to dynamically calculate the temperature at various depths within a geothermal well. Their model, validated in part with publicly available data from the Utah FORGE site, considers various cooling strategies to keep the temperature of drill bits and downhole measurement tools within their limits, and takes into account the effect of penetration (ROP) and hole-deepening in harder rock. Knowing the real-time temperature at any point in a well has two key benefits. First, the team can measure the effectiveness of cooling techniques, such as using surface mud chillers/coolers and drillpipe insulation. Second, they can better predict when and where equipment might begin to fail and extend the mean time between failures.
Equipment failure, specifically of drill bits, is the focus of RAPID senior research scientist Pradeep Ashok, Walker Department of Mechanical Engineering professor Dongmei “Maggie” Chen, and graduate research assistant Jian Chu. They have developed a model to figure out exactly why a bit has failed using simple 2D cell-phone images and videos taken directly at the well site.
“In the future, when a bit fails, the driller will pull the bit out of the well and just send in videos and photos. Our model will use that information to help them quickly make a decision about what to do next,” says Ashok. “Right now, those decisions aren’t made fast enough — and every hour that they do nothing or make the wrong decision costs more money.”
Van Oort, Chen and Ashok are also creating new models to monitor equipment wear in real time and predict failure before it happens. With graduate students Yifan Zhang and Dongyoung Yoon, they’ve designed models to detect and alert engineers to damaging vibrations, which intensify when drilling through harder rock, and predict the degradation or impending failure of the mud pumps that circulate hot fluid and ensure adequate pressure, lubrication and cooling. The latter technology has been successfully trialed by the Japanese industry consortium JOGMEC on geothermal wells in Japan.
Another model uses continuous 3D imaging to monitor rock cuttings and cavings coming out of the well that might indicate potential problems with well cleaning and borehole instability. A new AI model they have developed with graduate student Abraham Montes analyzes drilling patterns, wellbore geometry and fluid pressure to prevent the dreaded “stuck pipe” problem, a costly worst-case scenario that may necessitate cutting off the drillstring, side-tracking or abandoning the well, and starting over, losing considerable time and money in the process.
Essentially, the goal of all these models is to determine exactly when to better plan for drilling and make critical repairs or adjustments before catastrophic damage occurs. Real-time measurements, predictions and corrections not only make operations more efficient through saved time and money, but they also allow for continuous, fully automated (and safer) monitoring instead of the infrequent human-conducted monitoring commonly used in the field today.
Automation presents another opportunity: digital twinning. Once comprehensive, continuous data is collected from a drilling site, data analytics, machine learning (ML) and AI tools can be used to turn that information into a fully virtual twin of an individual well, or even an entire field of wells. With data from the digital twin, engineers can identify ways to optimize the drilling process, predict and solve problems, and prevent failures and accidents in real-time.
All of these advancements will go a long way toward making geothermal energy production economically viable, says van Oort. But it will take a village, as the saying goes.
“As petroleum engineers, we have the expertise in the subsurface well construction space, but this challenge can’t be solved by one discipline. It requires multidisciplinary interaction, involving not just petroleum engineering, but mechanical engineering, civil engineering, computational engineering, geology, hydrology, data analytics, ML and AI, and others to arrive at integral solutions.”
— Eric van Oort, professor, Hildebrand Department of Petroleum and Geosystems Engineering
With Maria Juenger, professor in the Fariborz Maseeh Department of Civil, Architectural and Environmental Engineering, van Oort and his team are studying geopolymers as an effective alternative to the ordinary Portland cement (OPC) traditionally used to cement wells. As temperatures and pressures increase — as they do in geothermal wells — OPC weakens, shrinks, bonds less effectively, and is more likely to allow drilling fluid leaks. Plus, it carries a relatively high carbon footprint. Cement manufacturing is the second-largest generator of carbon dioxide emissions worldwide. The team’s experiments indicate that certain lower-carbon geopolymers remain stable and strong even under the incredibly intense conditions of geothermal wells.
Geothermal Anywhere
The advancements being made by Sharma’s team and van Oort’s RAPID group will ultimately help drive down the cost of constructing and maintaining geothermal wells, which is crucial for geothermal energy to become a long-term and reliable addition to the global energy mix. Sharma’s and van Oort’s research also lends credence to a concept the National Renewable Energy Laboratory calls “geothermal anywhere.”
In a “geothermal anywhere” future, American military bases will run entirely on geothermal energy, eliminating their dependence on foreign energy sources. Communities will get hot water and heat from geothermal pumps right underneath their streets. Cities will run on electricity partially produced by geothermally powered turbines. Anywhere there is underground hot rock (and, remember, that’s everywhere), geothermal energy will be harvested.
Already, more than 30 countries get some amount of electricity from geothermal sources, with the U.S topping that list. In fact, just 20 miles from the Forty Acres, the largest-ever residential geothermal grid already exists in the Whisper Valley planned community — and it will eventually heat and cool about 7,500 homes when all six phases of the community’s master plan are completed.
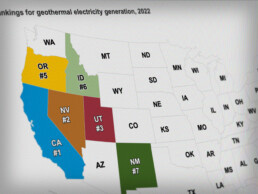
Leading states in geothermal-based electricity generation. details at eia.gov
“Until a few years back, I thought it would take maybe 15 or 20 years for geothermal to really get a good footing. But recently, the industry has seen quite a bit of success. Several commercial projects in non-traditional geothermal areas are in development. And in the next five to 10 years, activity will scale up significantly.”
— Pradeep Ashok, RAPID senior research scientist