By Nat Levy
Battery pioneer John Goodenough turned 100 last year, adding another life accomplishment to a list that continues to grow. The 2019 Nobel Prize winner and inventor of the lithium-ion battery has spent more than a third of those years advancing battery knowledge and technology at The University of Texas at Austin.
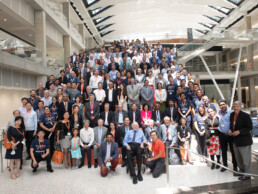
In that time, UT Austin has emerged as one of the leading hubs of battery and energy storage research in the world. It has grown beyond Goodenough, with more than 30 researchers — and dozens of students — across various schools, colleges and departments studying every aspect of battery science.
Altogether, UT Austin researchers study and create batteries from the earliest stages of mixing chemicals up to fabricating, testing them and bringing them to the market.
“We have such a wide range of topics covered in battery research, from molecules to miles, we do it all at UT,” said Venkat Subramanian, a professor in the Walker Department of Mechanical Engineering who focuses on modeling software to improve battery performance.
Battery Basics
Batteries come in all shapes and sizes, depending on what you’re using them for. The most common points of reference for most people are the AA and AAA batteries they put in remotes and many other household appliances.
Across all these different uses, from cars to laptops to energy storage, batteries are largely made up of the same core parts that perform key tasks. At the most basic level, a battery converts chemical energy into electrical energy that can supply power to electronics and other devices.
Chemical reactions in different parts of the battery produce electrons. And the flow of those electrons is what sends electricity to an external device.
Here is an explanation of the key building blocks of batteries:
- Cathode and anode: Together these are the positively/negatively charged electrodes that send out and receive electrons, galvanizing dueling chemical reactions called oxidation and reduction. In a typical household battery, the cathode and anode are the little bumps on the ends.
- The anode is the negative electrode that sends out electrons, a concept known as oxidation. And the cathode receives electrons, which is called reduction.
- Electrolyte: The medium that facilitates the transport of ions between the electrodes, creating a balance that ensures the reliability of the battery. Typically thought of as liquid, electrolytes can also be made of solid materials.
- The electrolyte physically separates the two core chemical reactions happening simultaneously in batteries. This separation is what allows batteries to be able to provide power to an external device without issue.
- Cell: A single anode and cathode separated by an electrolyte. Batteries can be as small as a single cell or include multiple cells.
It's a Material World
It’s no secret that batteries power a lot of things. The phones we scroll for too long. The laptops we sit in front of all day. And increasingly, the cars that get us around.
With all these uses, it’s easy to see why batteries are in high demand. One of the main thrusts in battery research today is figuring out how to lower the cost of making batteries, making them less expensive for the consumer.
In many ways, that comes down to materials. Extracting these scarce resources often requires extensive mining operations that are costly and take a heavy toll on the workers involved.
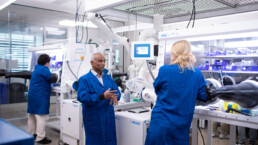
Mechanical engineering professor Arumugam Manthiram’s lab in the new Gary L. Thomas Energy Engineering Building (GLT).
The battery research community wants to find alternatives to some commonly used materials, like cobalt, that carry heavy human rights and environmental problems associated with their mining. The push to remove these materials from the battery mix is a top priority among researchers around the world right now.
“Cobalt is a problem today, nickel is a problem tomorrow, and lithium could become a problem a day after tomorrow,” said Arumugam Manthiram, a world-renowned battery expert and mechanical engineering professor who worked closely with Goodenough, first at Oxford and later at UT Austin.
The cost conundrum is one of several riddles facing battery researchers around the globe. The list of other attributes to balance and optimize is long, and that includes safety, sustainability, energy density (how long your phone battery lasts on a single charge), power (how long it takes to recharge) and life cycle.
The problem: improving in one aspect often means downgrading in another.
“You can have higher energy or better safety or lower cost, but not all of them together,” said David Mitlin, a mechanical engineering professor whose research focuses on alternative battery materials. “There’s always these tradeoffs between energy, safety, charging rate and cost.”
There’s a long-running joke in the battery community about finding the holy grail material that will solve all these problems. It will charge faster, without degrading and cost less than today’s materials without environmental complications.
They call it “unobtainium.”
However, there have been significant advances in battery materials in recent years. In 2020, Manthiram demonstrated a cobalt-free lithium-ion battery.
Manthiram and Mitlin in 2021 both published research that brings batteries that rely heavily on sodium — one of the most promising candidates to replace lithium and cobalt in batteries because of its sheer abundance — closer to reality. Mitlin’s team recently created a lithium battery that boasts improved energy storage and does it without an anode host.
“A lot of exciting things are happening right now, but everything is incremental,” Manthiram said. “There’s no magic technology that will change the world in the next one, two, or five years.”
Getting the Best Out of Batteries
Battery research at UT Austin runs the gamut — from atoms to real-life applications. Researchers are experimenting with new battery types, improving those that already exist, testing them to make sure they work and safety-checking them to avoid fires and explosions.
Hands-on experiments though are costly and take time. Many researchers at UT Austin leverage the super-computing power of the Texas Advanced Computing Center to use simulations to develop battery components.
Don Siegel’s work lies at the confluence of materials science and computation. He is the department chair of the Walker Department of Mechanical Engineering, and his work uses computational techniques to simulate materials down to the atomic level.
He mostly focuses on speculative battery technologies, such as the lithium-free batteries that other UT Austin researchers are working on. Simulations can test how dozens, or even hundreds of different materials will perform under a variety of circumstances.
Additionally, these techniques can be used to discover new materials that could lead to better performance than existing materials. Using trial and error in new material discovery through experiments can be time consuming. However, computer simulations can perform these tests much quicker.
“With computation we can predict how a new material will perform before it is synthesized and tested,” Siegel said. “That leads to major time savings. One could even file a patent application based on the computed predictions.”
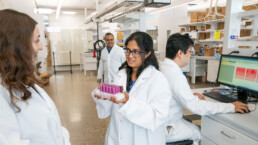
Professor Venkat Subramanian recently shifted his battery research lab to the new GLT building.
Modeling technology enables improved efficiency out of existing batteries. Subramanian’s group uses software and algorithms they developed to predict optimal conditions that allow a battery to not just meet but exceed expected benchmarks for key metrics, such as charging speed, cycle life and more.
Most batteries degrade over time. Smartphones are a great example — After two years, it feels like a phone’s battery life whittles away to almost nothing. That’s because degradation from so much use and so many charge/discharge cycles reduces the performance of the battery.
How a battery is used over time influences how long it will last before it starts to break down. Charging and discharging practices and how many things it has to do at once — think about a phone running a ton of apps in the background all the time — all play a role.
Because of the delicate chemistry and volatile nature of some of the materials in most batteries, you can’t just open them up and observe what’s happening. So, models and algorithms are among the best ways to optimize a battery.
“The model lets you figure out better than the best case for batteries,” Subramanian said.
Another path to that best case involves sensors. But once again, introducing new elements could disturb the highly sensitive and volatile reactions happening inside the battery.
Tanya Hutter, an assistant professor of mechanical engineering, is tackling this very problem. She is an expert at embedding sensors in biological tissues and other challenging environments, and she is expanding that focus to include batteries.
Hutter’s work is preliminary at this stage, though she has already begun working with Mitlin, Subramanian and other battery researchers at UT Austin. She plans to use optical fiber materials such as silica and polymers that are not chemically reactive, so they won’t disrupt the chemistry of the battery.
Real-time continuous sensing inside the battery cell could give a clearer picture of how various conditions affect the state of charge of a battery and monitor for any changes in chemistry.
“Instead of it being a black box, we want to embed sensors inside the battery and obtain accurate chemical and structural information at a desired location inside the battery cell. If we know more about the real-time changes in the battery’s chemistry, we can then gain an understanding of the underlying processes and use that knowledge to improve the technology, and even predict catastrophic events like fire or explosion,” said Hutter.
Mechanical engineering assistant professor Tanya Hutter’s work focuses on embedding sensors in challenging environments.
Safety First
For a short moment in time, six years ago, battery fires were top of mind across the world. That’s because of a series of incidents involving Samsung Galaxy Note 7 smartphones.
Eventually, experts found that the batteries’ design didn’t leave enough room for the electrodes. Batteries contain highly reactive elements, and any imperfections in manufacturing or moderate deviations in the battery environment can cause batteries to overheat and even explode.
At UT Austin, a group of researchers study fire and the many areas where it happens. This group, led by mechanical engineering professor Ofodike Ezekoye, studies battery fires, mitigations and solutions as part of its work.
For lithium-ion batteries, the main fire hazard comes from self-heating chemical reactions due to thermal instability of the battery materials. Fires can occur because of thermal runaway, a chain reaction within a battery cell when it gets too hot that can be very difficult to stop once it has started.
“Within these cells, you have the perfect recipe for disaster — fuel, oxygen and heat, all self-contained,” Ezekoye said.
A demonstration of a laptop battery exploding in professor Ofodike Ezekoye’s lab.
Thermal runaway creates a cycle of increased heat and temperature that build on each other. The increase in temperatures, up to more than 1600 degrees Fahrenheit can lead to high rates of gas generation from the battery and fires that are challenging to put out.
Like many technologies, safety was not the first concern in the development of lithium-ion batteries. But now safety is becoming an important aspect of building new batteries.
Ezekoye’s group is part of a large U.S. Department of Energy Advanced Research Projects Agency-Energy effort called EVs4ALL. The goal is to anticipate future safety risks in new batteries, or, “de-risking” materials. Together with materials researchers, Ezekoye and his team will make safety a centerpiece in the creation of next-generation battery technology.
“Let’s invest upfront in what the safety issues might be with future battery technology before we make this enormous investment in infrastructure and production,” Ezekoye said.
A Top Priority
Earlier this year, the Cockrell School’s newest state-of-the-art facility, the Gary L. Thomas Energy Engineering Building, opened its doors. Several battery research groups have already moved into the new building.
Mitlin and Subramanian are neighbors on the second floor of GLT. Siegel’s computational group is just a few doors down the hall, and Manthiram’s lab is one floor above.
On a recent visit, students point out where Subramanian’s lab ends and Mitlin’s begins, but no one is sure where that boundary truly lies. A lack of doors, walls and other dividers blurs the line between labs, and that’s by design.
The open layout encourages researchers to collaborate to spur innovation.
“It helps to be able to just walk out the door and find your collaborators or run into them in the hallway,” Siegel said. “It’s the best way to do it; put everyone in the same place and encourage them to share and work together by breaking down walls and blurring boundaries.”
And in the area of batteries that collaboration is growing. Many battery researchers at UT Austin are already working together, and as they get physically closer, it becomes even easier to collaborate.
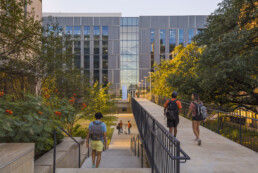
PHOTO CREDIT: ENNEAD ARCHITECTS
GLT is the latest sign of UT Austin’s commitment to being a leader in energy research, and batteries are a big part of that. In the same way college football teams hope to stand out with state-of-the-art practice facilities and weight rooms, research campuses can invest in modern lab facilities.
Newer investments like GLT and the recently opened Electron Microscopy Facility build on established programs and facilities such as Texas Materials Institute, Center for Electromechanics and the Texas Advanced Computing Center. In addition, later this year, a new expansive battery fabrication facility will open at UT Austin, making it easier to build batteries in-house after researchers put in the discovery and development work.
Numerous researchers we spoke to, some new to UT Austin, some who have been here for decades, cited facilities, collaboration possibilities and administrative commitment as major draws for faculty and students. Very few places can match UT Austin’s history of success of batteries, and that is a draw in of itself.
Overall, the researchers say, the vibes are strong for battery research at UT Austin, and that means we can expect to see more exciting discoveries on the way.
“Everybody is on the same page — that batteries are an important priority here — so it’s fairly joyous operating because you know the wind is at your sails,” Mitlin said.
To learn more about the more than two dozen battery researchers at UT, and everything they are working on, visit: https://batteries.engr.utexas.edu/